HYPERION’s objectives are:
Reliable quantification of climatic, hydrological, and atmospheric stressors employing quality-assessed numerical modeling results for selected CC scenarios in the historic areas under consideration, covering processes and interactions from the short-term to the long-term (10-60 years).
Multi-Hazard modeling covering single, contemporaneous (e.g., extreme temperature, humidity, wind, air pollutants) and cascading (mudflow/landslide after rain, etc.) hazards. Deterioration patterns and dose-response functions of building materials will be integrated in Heat, Air & Moisture (HAM) simulations
Analysis of bulding materials and deterioration processes
Implementation of a Hygro-Thermal (HT) simulation tool that considers the coupled HAM transport phenomena through structure’s elements under specific scenarios.
Improved prediction of Structural and Geotechnical (SG) safety risk of the structures through the use of simulators that exploit monitoring data from various sensors.
Environmental and material monitoring including state identification and damage diagnosis: novel Computer Vision (CV) and Machine Learning (ML) algorithms will be implemented to exploit sensors, such as visible spectrum cameras, hyper-/multi-spectral cameras, thermal/infrared/Ultra-Violet sensors, to get a precise inspection of CH sites.
MDesign of a Holistic Resilience Assesment Platform (HRAP) and a Decision-Support-System (DSS), enabling communities’ participation.
On-site Integration, Demonstration, and Validation of the HYPERION platform through case studies in Greece, Italy, Norway, and Spain.
Project Handbook
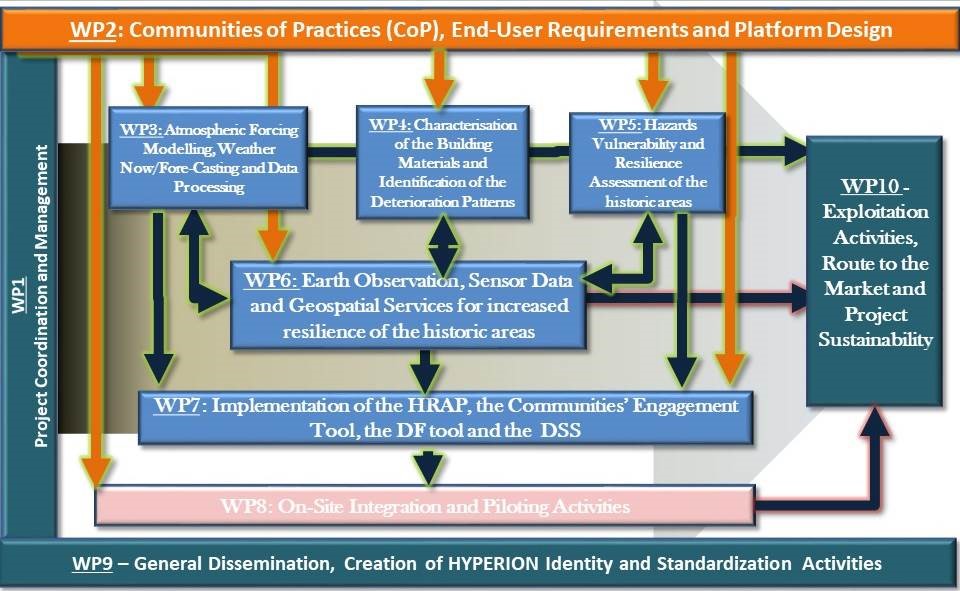
Analysis
- Scientific and Technical Objectives (STO):
Reliable quantification of climatic, hydrological and atmospheric stressors: we will employ quality-assessed numerical modelling results for selected CC scenarios in the historic areas under consideration, covering processes and interactions from the short-term to the long-term (10-60 years). These data will be used to estimate quantitative indicators for the potential impacts of CC on historic areas from the individual building to a regional level, including also aspects related to their aesthetics due to long-term exposure of the structures on air pollution and microclimatic conditions. Changes of both the average climate and the increase of the intensity and the frequency of extreme climatic/weather events will be considered. A Land Surface model will be used to account for the impact of climate and atmospheric composition on soil surface parameters, thereby quantifying the structural and thermo-physical impacts on the structural elements and operation. The high-resolution modelling effort will exploit existing sources of climate and air pollution data enriched with sensor data (on site) and enhance their added value through risk indicators for selected risk “hot-spots” (e.g., foundations, facades of buildings), introducing a risk modelling interface with our resilience assessment platform (STO-7).
Multi-Hazard modelling will cover single, cotemporaneous (e.g., extreme temperature, humidity, wind, air pollutants) and cascading (mudflow/landslide after rain, etc.) hazards. Inundation maps will be provided for specific catchments by using hydrological modelling for various precipitation capacities, while seismic hazard will be quantified in terms of seismic intensity levels (peak ground acceleration, spectral estimates, and surface faulting deformations) and their spatial/temporal distribution for the historic area, by using stochastic modelling approaches (probabilistic seismic hazard analysis). HYPERION aims to provide input for the relevant regulatory framework, (e.g., Eurocode 1), on the load models for climatic actions. Data-based calibration of these models will be done at case study level, and methodologies for evolution of these load models to take into account CC will be proposed.
Analysis of building materials and deterioration processes: deterioration patterns and dose-response functions of building materials to be integrated in Heat, Air & Moisture (HAM) simulations will be achieved for:
– Classification of various building materials and damage assessment at demonstration sites, physical-mechanical characterisation of fresh, unaltered samples.
– Identification of critical first order factors that are not currently considered in the available recession models, and their measurement at CH-scale:
- microclimatic factors (temperature, relative humidity, wind direction and turbulence at material surface, runoff, dry fallout, sunlight irradiation, surface orientation).
- Petrographic and physical-mechanical features of building materials: mineral composition, texture, grain size, pore structure;
– Deterioration of physical-mechanical properties of materials. Predicting CH vulnerability requires knowledge on the decline of physical-mechanical properties of building materials with deterioration. The following tasks will be completed:
- Accelerated aging tests in climate testing chamber and evaluation of stone recession by confocal microscopy;
- Assessment of physical-mechanical decline of these materials;
- Exposure at demonstration sites, analysis of surface topography evolution by portable confocal profilometry, comparison with aging tests and topography measurements on monument surface.
- Identification of deterioration morphologies and processes;
– Refinement of damage and dose-response functions:
- Correlation among microclimate time series, stone petrographic and textural features and recession rate;
- Formulation of material-specific dose-response functions combining results from petrographic characterisation and stone recession measurements.
- Systematic deviation between climate time series and surface microclimate conditions to back-reconstruct microclimate time series.
– Effect of extreme events & environmental aging processes on deterioration of building materials:
- Identify gauge height of historical flooding from sensors and assess decay related to floods;
- In coastal areas, map the distribution of salt efflorescence from rising damp and marine aerosols, reconstruct air circulation in an urban canyon environment and determine control on salt distribution at buildings;
- Map and incorporate the cumulative effect of seismic sequences on historical structures;
- Predict effect of increased frequency of extreme events.
Implementation of a Hygro-Thermal (HT) simulation tool that considers the coupled HAM transport phenomena through structure’s elements under specific scenarios. The following actions will be implemented:
- Analysis of CC risk scenarios in 1D, 2D and 3D spatial resolution and assessment of CC impacts on acceleration of materials and elements degradation using the developed HAM model.
- Characterization of microclimate, both exterior and interior, using Computational Fluid Dynamics (CFD);
- Dynamic HAM (d-HAM) modelling of the hygrothermal performance of building materials that integrates boundary conditions obtained through transient CFD models;
- Enrich validated databases of hygric and thermal building material properties to be used in HAM simulations.
- Quantification of the pace and magnitude of the change of material properties through comparative analysis between modern local materials and in-site materials, both exposed to the same local climate loads;
- Validation of d-HAM models of materials’ properties using time-series experimental data;
Improved prediction of Structural and Geotechnical (SG) safety risk of the structures through the use of simulators that exploit monitoring data from various sensors. Along with the expert knowledge of partners specializing on SG engineering and on materials’ deterioration, HYPERION will assess the current condition of structural, non-structural and content components of characteristic archetype buildings in the historic area.
Τhese detailed models will be leveraged
- to validate simplified surrogate numerical models or reduced-order physical models,
- achieve accurate pre-event and near-real-time (n-RT) post-event assessment of the impact of the climate pressure and geo-hazards,
- define related damage/vulnerability functions and capacity thresholds of the aging structure,
- optimise any reconstruction or retrofitting actions and finally evaluate the response of the structure in the future, for a large number of hazards scenarios with/without the proposed adaptation and mitigation measures.
Environmental and material monitoring including state identification and damage diagnosis: novel Computer Vision (CV) and Machine Learning (ML) algorithms will be implemented to exploit sensors, such as visible spectrum cameras, hyper-/multi-spectral cameras, thermal/infrared/Ultra-Violet sensors, mounted on vehicles and drones to get a precise inspection of CH sites. Main outcomes will be:
- 3D capturing of wider area, ground based imaging and 3D reconstruction;
- Damage assessment and structure deformation maps (internal/ external walls, facades/roofs and envelopes), surface material classification and degradation analysis and contour diagrams for the temperature profile;
- Novel methodologies for deformation maps, ML-based algorithms for the assessment of land cover changes in the broader area, and overall estimation of the environmental condition of the CH site (big picture: flooding, sea-level rise, ditches’ overflows, soil moisture, etc.).
- spatio-temporal 4D (3D plus time) maps assessing the temporal damages of sites and the impact of the climate on its conditions;
- integrated conventional sensors (e.g., environmental and structural) and deployment of low-cost microclimate-stations (wind-speed, air humidity, soil moisture and temperature) and smart tags (air humidity, temperature, vibrations).
Design of a Holistic Resilience Assessment Platform (HRAP) and a Decision-Support-System (DSS), enabling communities’ participation. HRAP will allow the integration of various analysis, modelling tools and damage/vulnerability functions, hence incorporating information from various sources (literature, surveys, satellite, etc.) with different levels of granularity (building/block/regional level) together with the associated uncertainties.
All these tools will be built on a Geographic Information System (GIS), interfaced with existing open-source hazard assessment software (e.g., OpenQuake) and network simulators (e.g., EPANET, traffic simulators), and chained to socioeconomic impact analysis tools to produce both quantitative and qualitative loss estimations (e.g. financial loss estimation, reputation impact, morale impact etc.) in order to develop an end-to-end simulation platform enabling the running of any number of different “what-if” scenarios and offer:
- Comprehensive risk and impact assessment of hazards on the structural/non-structural/content components, such as stone and masonry walls, sculptures, frescoes, paintings, etc.;
- Testing of various risk management approaches, plans, strategies, countermeasures and adaptations for the selected structures;
- Understanding the sensitivity of system assets, structures, and services to the selected types of hazards;
- Understanding interdependency due to cascading events, i.e. failure to energy or transport network, interrupting other business;
- Setting up risk-based response strategies adapted to specific scenarios and defining efficient standard response procedures,
- Assessing and quantifying the overall resilience of the historic area with a holistic quantitative approach.
- Setting up contingencies and pre-qualified responses for critical supply chain operations and disruption in order to promote end-to-end resiliency,
- Support the community-based participatory environment in the platform (link with the existing social platform –PLUGGY- for increased CH participation and awareness and build Application Programming Interfaces (APIs) for social media);
- Plan and budget for focused structural rehabilitation efforts of buildings and gauge the effect of associated policies to improve area-wide resilience;
- Introducing local community load balancing models so as to ensure survival of the surrounding residential and business areas;
- Estimating fair insurance rates, pricing CAT-bonds and optimizing their triggers (i.e., the threshold of a third-party verified sensor reading that contractually signals the release of funds) to finance a rapid bounce-back;
- Evaluating the impact over time right after a disruptive event, in order to facilitate faster response and minimize financial losses,
- Evaluating and assessing the Maximum Acceptable Damage (MAD) that a CH site can afford before being considered as a total loss.
HRAP aims to integrate all the hazard and impact assessment tools and modelling data (climatic, SG and HT) in order to support decisions at strategic, tactical and operational level.
On-site Integration, Demonstration and Validation of the HYPERION platform through case studies in Greece, Italy, Norway and Spain.
The main focus will be on:
- assessing damages on materials/structures and finding tailor-made solutions to ensure their conservation and improved resilience;
- testing the project outcomes in real-scale critical structures and the CH site. The cost, benefit and the time of the proposed system and incurred procedures will be compared to the manual or non-automated procedures/means up to the initiation of the project.
Provision of a Handbook to include:
- technical information on sustainable reconstruction of historic areas,
- proper adaptive response strategies for CC and other hazards scenarios,
- post-disaster reconstruction examples,
- practical checklists and references to assist practitioners, field-workers, cities and cultural authorities, etc. in better decision making,
- recovery requirements for various sectors,
- information on financial tools to mitigate risk, including a novel set of CH-area-specific insurance-linked securities (e.g., CAT-bonds) designed to cover different degrees of extreme CC and non-CC event severity and
- guidelines and techniques to encourage, facilitate, and develop bespoke reciprocal agreements between same type of businesses for timely service recovery.